Chap 11 HW
1. Vocabulary
1. Muscle Origin and Insertion
- Origin: The proximal attachment point of a muscle, typically more stable during contraction.
- Insertion: The distal attachment point of a muscle, which typically moves more during contraction.
2. Muscle Fiber Type (Multiple Methods for Determining This)
- Histochemical staining: Uses enzymes like myosin ATPase to distinguish between slow (Type I) and fast (Type II) fibers.
- Immunohistochemistry: Uses antibodies to detect specific myosin heavy chain (MHC) isoforms (e.g., MHC-I, MHC-IIa, MHC-IIx).
- Electrophoresis: Separates myosin isoforms by molecular weight.
- Functional properties: Measures contraction speed, fatigue resistance, and oxidative vs. glycolytic capacity.
3. Pennation Angle
- The angle at which muscle fibers attach to the tendon. Higher pennation angles allow more fibers to fit into a given volume, increasing force production but reducing shortening velocity.
4. Cross-Sectional Area (CSA)
- The area of a muscle perpendicular to its longitudinal axis. Often measured via imaging (MRI, ultrasound) and relates to muscle size but not necessarily force production.
5. Physiological Cross-Sectional Area (PCSA)
- The total cross-sectional area of all muscle fibers, accounting for pennation angle. PCSA is directly proportional to a muscle’s maximum force-generating capacity.
6. Passive Tension
- The force generated by a muscle when stretched beyond its resting length, due to elastic properties of connective tissue (e.g., titin, collagen) without active contraction.
7. Active Tension
- The force produced by a muscle when it contracts, generated by actin-myosin cross-bridge cycling within sarcomeres. Depends on factors like neural activation, fiber type, and muscle length.
2. Refresh Your Physiology
When an action potential reaches the end of a motor neuron, calcium enters and triggers the release of acetylcholine (ACh) into the synaptic cleft. ACh binds to receptors on the muscle fiber, causing depolarization and an action potential in the muscle.
This muscle action potential travels into the T-tubules, activating receptors that open calcium channels in the sarcoplasmic reticulum. Calcium floods into the sarcoplasm, binding to troponin and moving tropomyosin to expose actin’s binding sites.
Myosin heads, already energized by ATP, attach to actin, forming cross-bridges. The myosin heads then pivot (the power stroke), pulling actin filaments inward and creating muscle tension.
For myosin to detach and reset, ATP binds to it, breaking the cross-bridge. ATP is then split into ADP and phosphate, re-cocking the myosin head so the cycle can repeat. Without ATP, the muscle stays locked in contraction.
3. Add to Your Physiology
Residual force enhancement refers to the increased steady-state force a muscle can produce after being actively stretched compared to the force generated during a purely isometric contraction at the same length. This means a muscle holds more tension after being stretched while activated than if it had simply contracted at that length without prior stretching.
The mechanisms behind this phenomenon are not fully understood but likely involve two key factors. First, passive elastic elements like titin may stiffen during active stretching, contributing extra force. Second, uneven sarcomere lengths and increased cross-bridge attachments after stretching may lead to greater force retention. Some research suggests that calcium sensitivity also plays a role, with stretched muscle fibers maintaining higher force even at the same calcium levels.
In sports and rehabilitation, this concept could help optimize strength training and injury recovery. Athletes might use controlled active stretching (like eccentric exercises) to enhance muscle force output, improving performance in explosive movements. Clinically, therapists could apply slow, loaded stretching to help patients regain strength after injuries, taking advantage of residual force enhancement to rebuild muscle capacity more effectively. Understanding this effect could also refine post-surgical rehab protocols, ensuring muscles regain maximal function.
4. Draw This
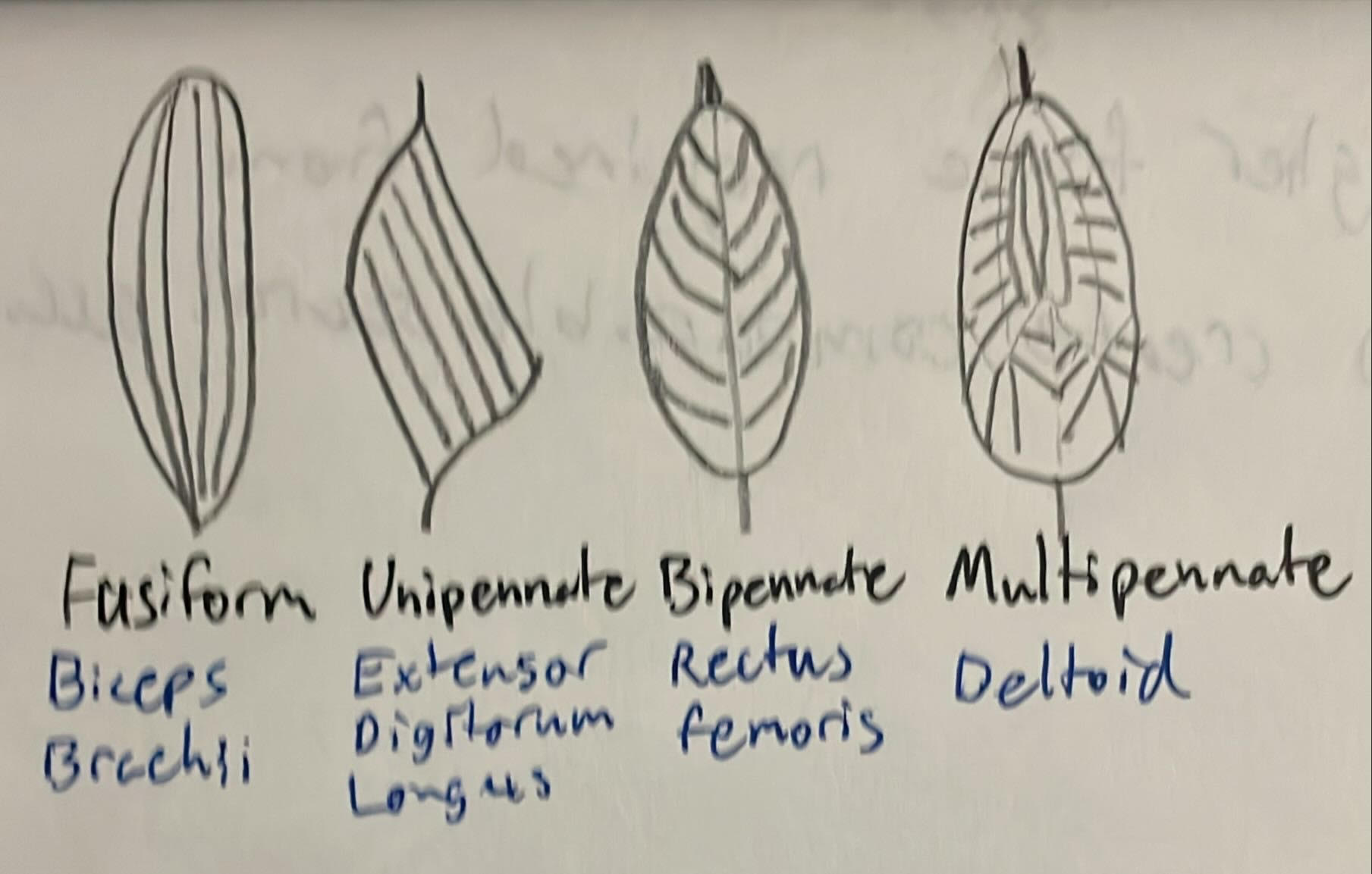
5. Plot this
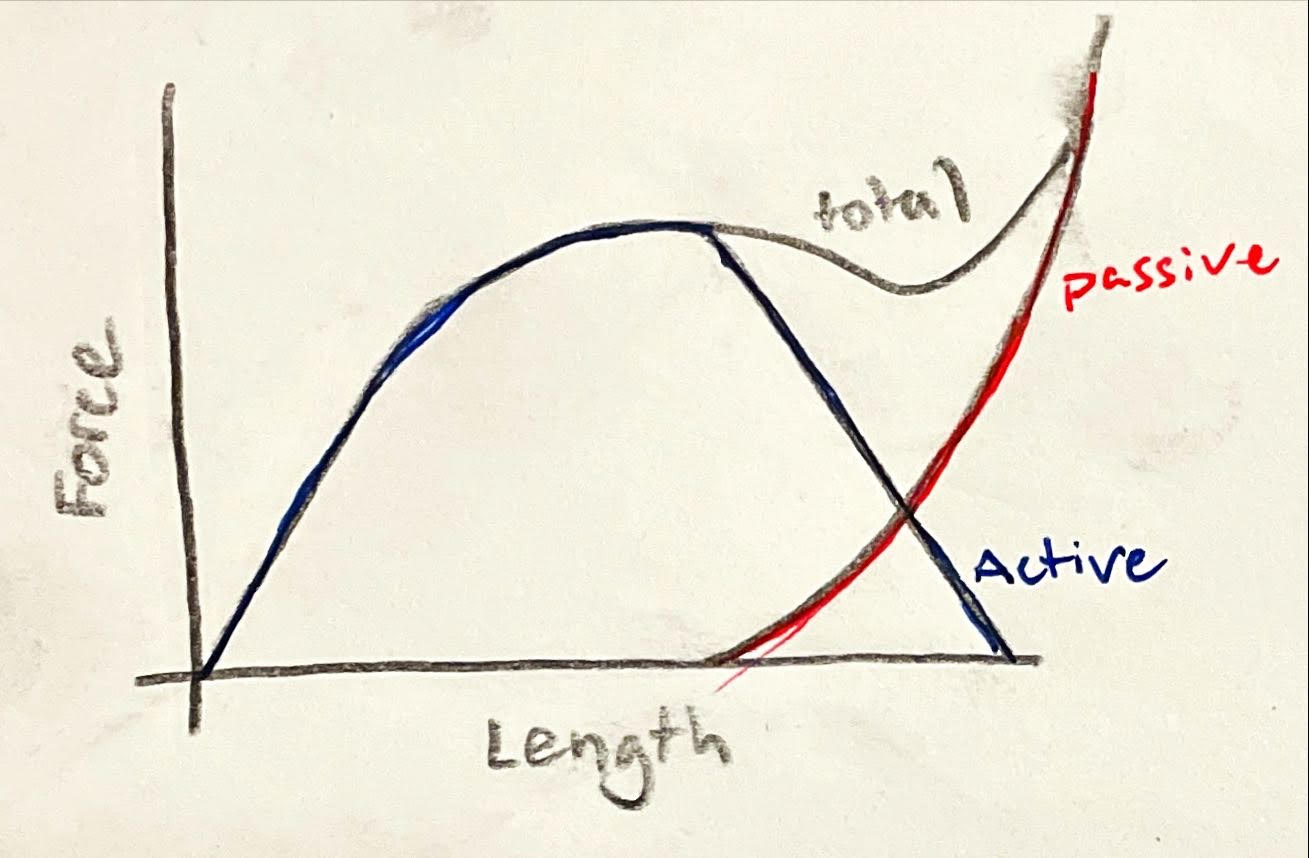
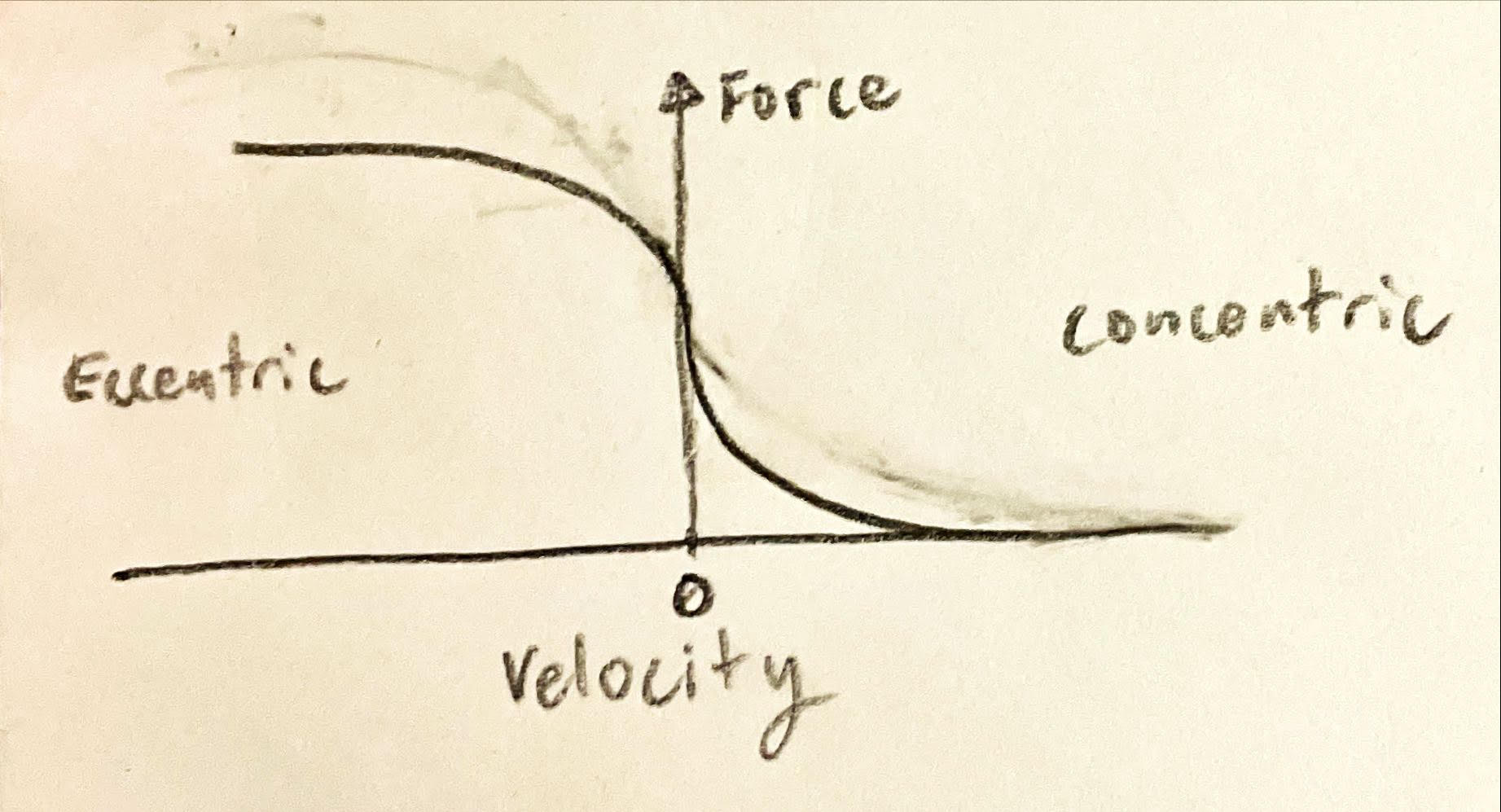
6. Mathematically Describing Muscles
A.
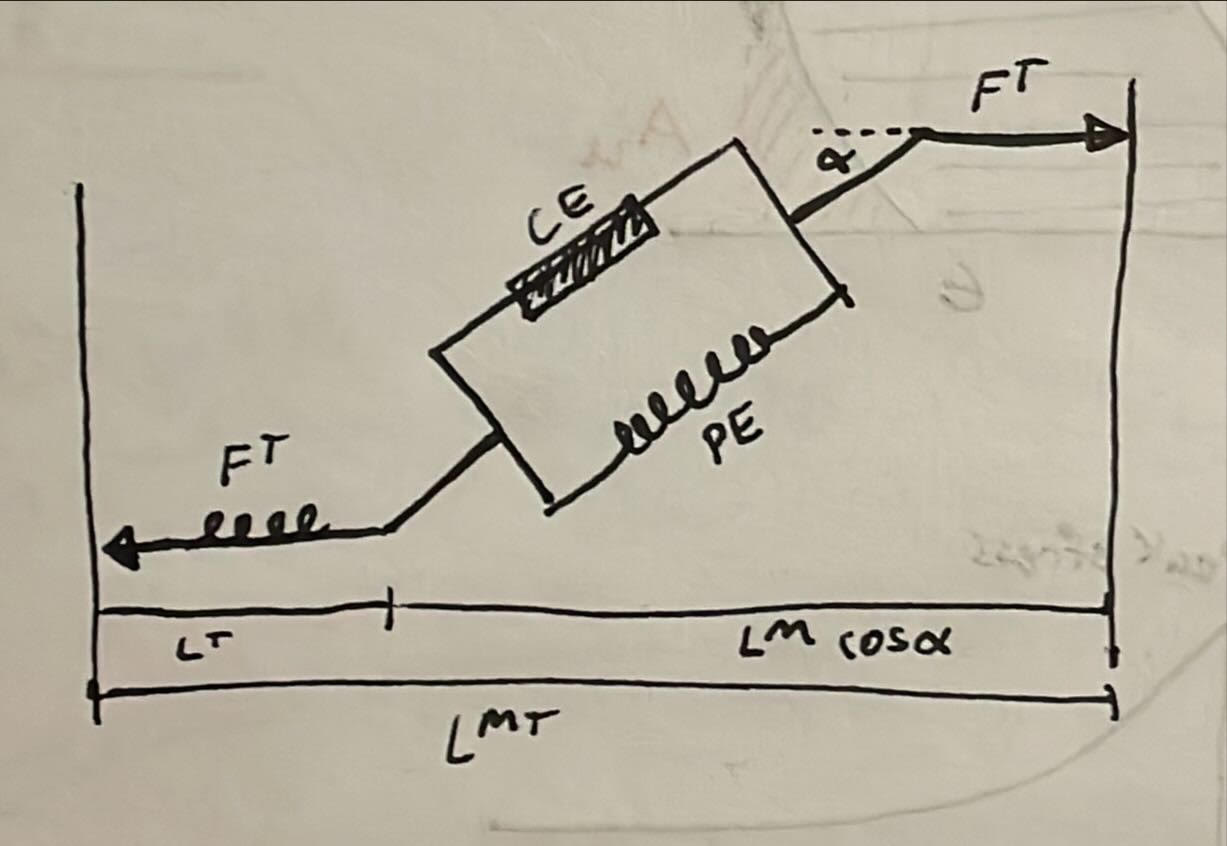
B.
$ L^{MT} $ — Muscle-Tendon Length
The total length from the origin of the tendon to the insertion point of the muscle-tendon unit. It includes both the muscle and the tendon.$ L^T $ — Tendon Length
The length of the tendon portion alone, which connects the muscle to the bone and transmits force.$ L^M $ — Muscle Fiber Length
The length of the muscle fibers (also called the contractile part of the muscle).$ \alpha $ — Pennation Angle
The angle between the muscle fibers and the line of action of the tendon. This affects how much of the muscle fiber force contributes to the tendon force.$\text{CE}$ — Contractile Element
This represents the active component of the muscle. It generates force when the muscle is stimulated by neural input.$\text{PE}$ — Parallel Elastic Element
This represents the passive elasticity of the muscle, which resists stretch and contributes to force passively (i.e., without activation). It mimics the behavior of muscle tissue when stretched.
References
Week 13 Lecture, Montana State University, Department of Food Systems, Nutrition, & Kinesiology, Fall 2024.
Lo, A. (n.d.). Squeakyspacebar.Github.Io. Retrieved April 24, 2025, from https://squeakyspacebar.github.io/kinesis-doc/01-basics/#installation-and-setup